Research
Context of CeeD studies
Diabetes is a chronic disease occurring when the pancreas can no longer secrete insulin or when the insulin-sensitive organs can no longer respond to insulin signal, impacting glucose homeostasis. Around 537 million people were living with diabetes in 2021 and these figures are constantly growing with a projection of 783 million diabetics in 2045, representing 1 in 8 adults.
Source: IDF
Glucose homeostasis
Glucose homeostasis is of high importance for body normal function. Pancreas endocrine function is the main controller of the glucose blood level [1]. Pancreas is the central organ controlling glucose homeostasis through its islets of Langerhans containing cells regulating hypo- and hyper-glycaemic hormones [1]. Β cells secrete insulin, a hypo-glycaemic hormone, when high blood glucose level is detected and α cells produce glucagon, a hyper-glycaemic hormone, when hypoglycaemia is detected. Insulin, by binding onto its receptors on different insulin-sensitive cells, activates the transport of glucose from the blood circulation to cells, where it is stored and used according to energy needs or episode of hypo-glycaemia [1]. Indeed, glucagon secretion induces the glucose release from cells to the blood.
Integrative physiology: organ crosstalk
As previously mentioned, insulin enables the transport of glucose from blood circulation to insulin-sensitive cells. Indeed, in addition to the pancreas, a crosstalk communication between it and other organs, called insulin-sensitive organs, has been highlighted [2]. Today, the implication of pancreas, adipose tissue, liver, brain, muscle, bones and intestine in glucose homeostasis has been described. However, like the hidden face of an iceberg, new interactions in this crosstalk communication between these different insulin-sensitive organs are discovered every day, highlighting the complexity of diabetes [2].
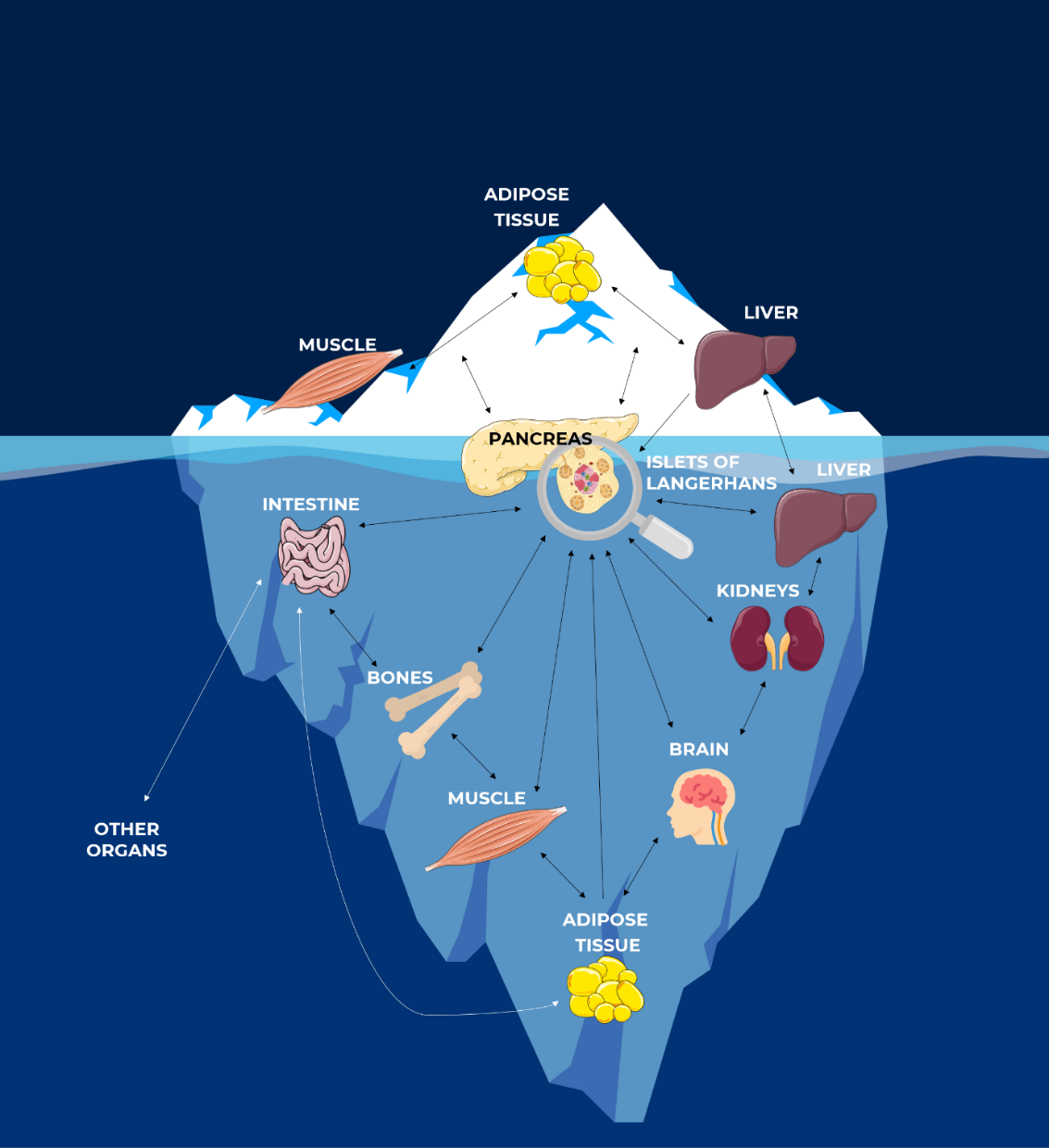
The Hidden Face of Iceberg: Crosstalk Communications Between Islets Cells and Insulin Target Tissue ©CeeD
Implication and complications
of the muscle, liver, adipose tissue and kidneys
At CeeD, we particularly focus on the implication of the muscle, liver, adipose tissue and kidneys in glucose homeostasis but also on the complications on these organs generated by the diabetes.
Muscle
Skeletal muscle accounts for about 40% of the human body weight, thus representing the largest organ in the body [3]. The main function of skeletal muscle is to contract for posture control and movement production but it also plays a critical role in whole body glucose homeostasis. Indeed, it is responsible for over 80% of insulin-stimulated glucose uptake [3]. Once in skeletal muscle cells, glucose is used to provide energy for muscle contraction and the excess of glucose is stored in the form of glycogen through glycogenogenesis [4]. This reserve of substrates will be quickly available when skeletal muscle needs more energy (exercise, fasting) [4].
Adipose tissue
The principal role of adipose tissue is to store energy to constitute an important fuel reservoir for the body. After a meal, increased in insulin secretion leads to glucose and free fatty acids (FFA) uptake in adipose tissue in which they are stored as triglycerides (TG) [5]. During post absorptive phase, TG are hydrolysed by lipolysis and so FFA are released to ensure sufficient energy status [5].
Adipose tissue has long been considered a mere storage tissue but it is also an important endocrine organ. It secretes a lot of bioactive factors including proteins (adipokines), lipids (lipokines) and extracellular vesicles involved in many physiological mechanisms such as insulin sensitivity and secretion, appetite or vascular tone control [5].
Liver
The liver has an important role in the regulation of glucose homeostasis. In the same way as the muscle, the liver, in response to insulin, uptakes the glucose and stores it in the form of glycogen through glycogenogenesis [6].
When the glycaemia is low, and in response to glucagon, the liver has the capacity to release glucose back to the blood circulation, through glycogenolysis, which converts stock of glycogen to glucose [7]. After a longer period of fasting, when the stock of glycogen is exhausted, the liver has the capacity to synthesize and release glucose to the blood circulation from non-carbohydrate compounds such as amino acids [7].
Kidneys
The main role of the kidneys is to filter the plasma, which means to reabsorb the substances that should be needed by the organism, such as the glucose, and to eliminate the other substances in the urine [8]. This is carried out by the nephrons, kidneys’ base unit, composed of filter part, the glomeruli, connected to a tubular portion reabsorbing the filtrate. For a long time, kidneys were not considered as source of glucose, however, kidneys are still organs involved in glucose metabolism [8]:
- Glucose storage by the kidney according to their needs;
- Glucose release in the blood circulation through glycogenolysis and gluconeogenesis in response to energy needs;
- Glucose reabsorption from kidneys to the blood circulation.
Generally, the kidneys reabsorb the maximum of filtrated glucose as possible through the membrane glucose transporters (GLUTs: passive transport; SGLTs: active transport) present on the proximal tubes. This reabsorbed glucose comes from the filtrated plasma in the nephrons. When the capacity of these transporter is reached, the glucose is then eliminated in the urine [8].
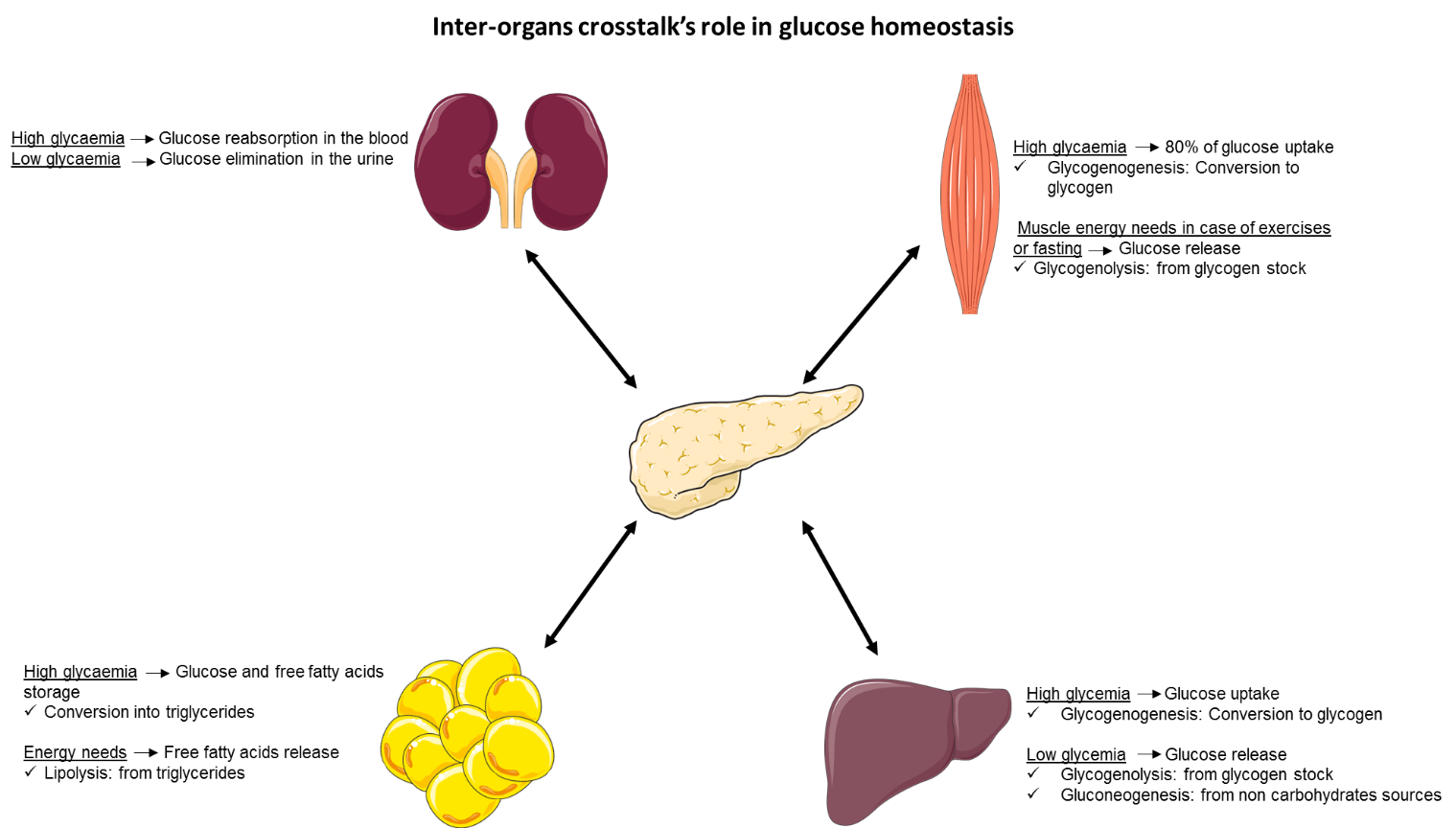
Diabetes
Two principal types of diabetes exist: type-1 diabetes and type-2 diabetes.
Type-1 diabetes (T1D): Type-1 diabetes is induced following β cell destruction by T cells. Thus, insulin secretion is impaired and glucose is no more uptake into insulin-sensitive organ cells. Glucose homeostasis is then no more controlled [9].
Type-2 diabetes (T2D):
First hallmark of T2D is insulin resistance occurring in insulin-sensitive organs. Those organs do no more respond to insulin signal, thus glycaemia stays high and β cells will, in response, produce more and more insulin inducing the second hallmark: β cells exhaustion. Finally, this β cell exhaustion is responsible of a lesser insulin secretion and so of type-2 diabetes [9].
Effects of physical exercise on diabetes
The first main hygieno-dietetic measures prescribed for diabetic patients are food rebalancing and physical exercise. The role of skeletal muscle in glucose homeostasis is also mediated by its endocrine function [10]. It is capable to secrete a lot of cytokines called myokines, particularly during exercise. These molecules can have beneficial impact locally but also in distant tissues, such as pancreas, adipose tissue or liver [10].
Thus, we focused on muscle secretome during physical exercise and notably on myokines. Indeed, it has been shown that muscle secretome is modified during physical exercise versus sedentary activities. Thus, the laboratory highlights that the muscle secretome is also dependent on the muscle type: endurance (such as quadriceps) or resistant muscle (such as triceps).
This muscle secretome is composed of:
- Myokines: cytokines secreted in response to physical exercise [11],
- Exosomes: vesicles containing proteins and genetic materials [12].
[1] P. V. Röder, B. Wu, Y. Liu, et W. Han, « Pancreatic regulation of glucose homeostasis », Exp Mol Med, vol. 48, no 3, p. e219, mars 2016, doi: 10.1038/emm.2016.6.
[2] A. Langlois, A. Dumond, J. Vion, M. Pinget, et K. Bouzakri, « Crosstalk Communications Between Islets Cells and Insulin Target Tissue: The Hidden Face of Iceberg », Front Endocrinol (Lausanne), vol. 13, p. 836344, 2022, doi: 10.3389/fendo.2022.836344.
[3] K. E. Merz et D. C. Thurmond, « Role of Skeletal Muscle in Insulin Resistance and Glucose Uptake », Compr Physiol, vol. 10, no 3, p. 785‑809, juill. 2020, doi: 10.1002/cphy.c190029.
[4] E. A. Richter et M. Hargreaves, « Exercise, GLUT4, and Skeletal Muscle Glucose Uptake », Physiological Reviews, vol. 93, no 3, p. 993‑1017, juill. 2013, doi: 10.1152/physrev.00038.2012.
[5] L. Luo et M. Liu, « Adipose tissue in control of metabolism », Journal of Endocrinology, vol. 231, no 3, p. R77‑R99, déc. 2016, doi: 10.1530/JOE-16-0211.
[6] H.-S. Han, G. Kang, J. S. Kim, B. H. Choi, et S.-H. Koo, « Regulation of glucose metabolism from a liver-centric perspective », Exp Mol Med, vol. 48, no 3, p. e218, mars 2016, doi: 10.1038/emm.2015.122.
[7] M. C. Petersen, D. F. Vatner, et G. I. Shulman, « Regulation of hepatic glucose metabolism in health and disease », Nat Rev Endocrinol, vol. 13, no 10, p. 572‑587, oct. 2017, doi: 10.1038/nrendo.2017.80.
[8] P. Curtis L. Triplitt CDE, « Understanding the Kidneys’ Role in Blood Glucose Regulation », Supplements and Featured Publications, vol. 18, no 1 Suppl, avr. 2012, Consulté le: 10 mars 2022. [En ligne]. Disponible sur: https://www.ajmc.com/view/ace005_12jan_triplitt_s11
[9] A. Petersmann et al., « Definition, Classification and Diagnosis of Diabetes Mellitus », Exp Clin Endocrinol Diabetes, vol. 127, no S 01, p. S1‑S7, déc. 2019, doi: 10.1055/a-1018-9078.
[10] M. L. Mizgier, R. Fernández-Verdejo, J. Cherfan, M. Pinget, K. Bouzakri, et J. E. Galgani, « Insights on the Role of Putative Muscle-Derived Factors on Pancreatic Beta Cell Function », Front. Physiol., vol. 10, p. 1024, août 2019, doi: 10.3389/fphys.2019.01024.
[11] K. Bouzakri, P. Plomgaard, T. Berney, M. Y. Donath, B. K. Pedersen, et P. A. Halban, « Bimodal Effect on Pancreatic β-Cells of Secretory Products From Normal or Insulin-Resistant Human Skeletal Muscle », Diabetes, vol. 60, no 4, p. 1111‑1121, avr. 2011, doi: 10.2337/db10-1178.
[12] S. Rome, A. Forterre, M. L. Mizgier, et K. Bouzakri, « Skeletal Muscle-Released Extracellular Vesicles: State of the Art », Front Physiol, vol. 10, p. 929, 2019, doi: 10.3389/fphys.2019.00929.